Andrey ZHUK
Deputy director of the JIT RAS,
D. Tech. Sc, Professor
e-mail: 666zhuk@ihed.ras.ru
Nikolay NOVIKOV
Leading Researcher of the JIT RAS,
D. Tech. Sc., Professor
e-mail: novikov_nl@ntc-power.ru
Alexander NOVIKOV
Research Associate of the JIT RAS
e-mail: novikov@energo365.ru
Viktor FROLOV
Leading Design Engineer of STC FGC UES
e-mail: Frolov_VD@ntc-power.ru
Introduction
“I am convinced that the development of hydrogen energy is the strategic future of the whole world,” wrote Vladimir Nakoryakov, Member of the Russian Academy of Sciences, back in 2009. The term “hydrogen energy” has gained immense popularity in the world of science, economics and politics in the last ten years. It refers to an industry based on the use of hydrogen as a means for storing, transporting, producing and consuming energy.
Although it is the most abundant element on the planet, hydrogen is virtually non-existent in its pure form in nature. It needs to be produced and converted to obtain its final consumer form. Both entail the necessary costs. More than 90% of the final energy comes from fossil hydrocarbon fuels and the carbon is still converted to CO2, so that when the final clean product is obtained and then used, the overall problem of reducing emissions remains unsolved. It only moves geographically. In places where pure hydrogen is produced, emissions are slightly increased as compared to the use of traditional liquid and gaseous fuels, but the clean product gives off fewer emissions at the consumer.
In the popular literature, hydrogen energy is sometimes contrasted with “hydrocarbon.” But hydrogen energy merely complements oil, nuclear or “renewable” energy – it is not a new source of energy in and of itself. In other words, it’s a way to make the most efficient use of available energy sources, to increase the efficiency of their use or to obtain other advantages. Hydrogen is a secondary energy carrier, but it deserves special attention as the most capacious and environmentally-friendly energy carrier of all existing chemical substances because the only product of its reaction is water. The heat of hydrogen combustion is 142 megajoules per kilogram, while that of natural gas is 28 to 46 megajoules. Since hydrogen can be widely used not only in energy but also in the chemical industry, it would be more correct to use the concept of “hydrogen economy,” which includes:
- production of hydrogen from water using non-renewable energy sources (hydrocarbons, atomic energy, thermonuclear energy);
- hydrogen production using renewable energy sources (sun, wind, hydropower, tidal energy, biomass, etc.);
- production of hydrogen from natural gas.
Hydrogen is not an energy source, and its generation is a means for converting the other energy resources into chemical energy in the form of stored pure hydrogen, which can subsequently be used in the oxidation of H2. In fact, a tank or other storage device for hydrogen is a technical device for converting natural gas with a utilization efficiency of 72%. This means that 28% of the energy contained in natural gas – methane, is lost, in addition to the energy that is used to extract and transport natural gas to the hydrogen plant. Only 4% of hydrogen is obtained from water by electrolysis. The cost of obtaining hydrogen from water (by various types of electrolysis) is 3-6 times higher than the cost of obtaining hydrogen from natural gas.
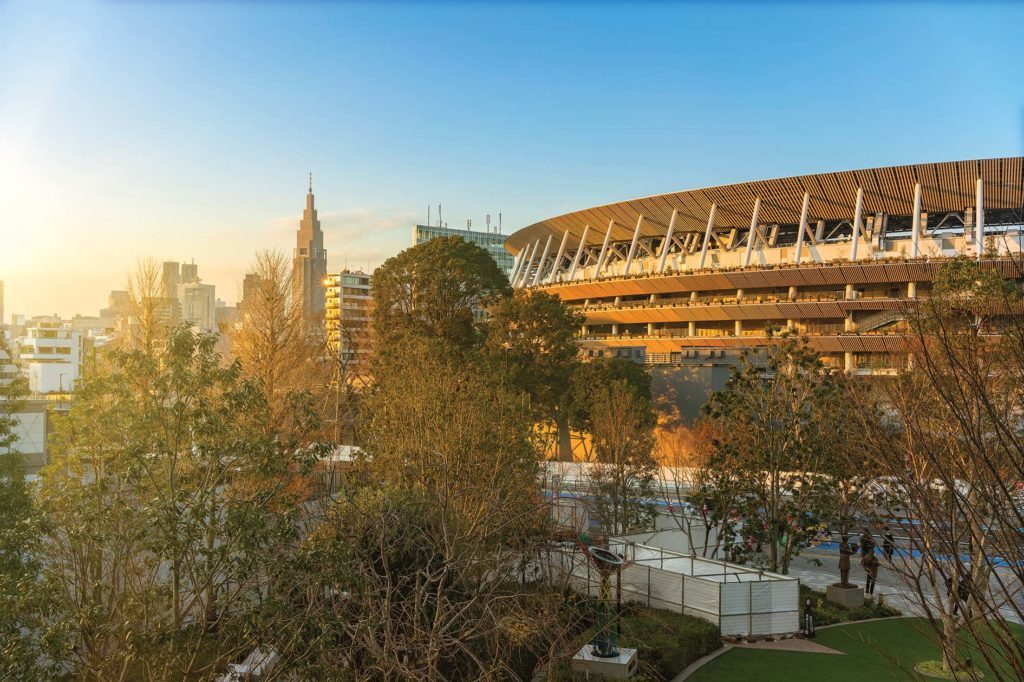
Source: kuremo / Depositphotos.com
As outlined in the Strategic Roadmap for Hydrogen and Fuel Cells, Japan’s Ministry of Energy, Trade and Industry (METI) [1, 2] is coordinating a long-term strategy to accelerate the adoption of hydrogen fuel cells and hydrogen power plants. METI expects Japan’s annual hydrogen consumption to grow from 4,000 tons in 2020 to 300,000 tons by 2030 and 5-10 million tons by 2050. The hydrogen price expected by METI is $3 for 1 kg of hydrogen by 2030 (equivalent to $0.8/L of gasoline), and by 2050, the price will drop to $2 for 1 kg of hydrogen ($0.5/L of gasoline). Prices for imported hydrogen in Japan are currently about $10 per 1 kg of hydrogen ($2.6/ liter of gasoline). This means that if by 2030, oil prices do not fall to $30-40 per barrel, hydrogen fuel will be quite competitive.
Depending on the primary type of energy used in the production of Н2, different “color” types of hydrogen are distinguished: “green” (RES energy for water electrolysis), “yellow” (atomic energy for water electrolysis), “gray” (production from methane by steam reforming), “blue” (production from methane by means of steam reforming using СО2 storage systems)
Even by 2070, the International Energy Agency predicts that energy will account for only about fourteen percent of the world’s total hydrogen consumption. Hydrogen is attractive due to its high energy capacity: three to four times more than that of natural gas. The use of hydrogen as a fuel has not yet become widespread, but many countries and companies are betting on it, since this energy resource, in combination with renewable energy sources, makes it possible to produce energy with zero emissions. It is the transport sector where a significant spread of this energy carrier is expected. If the requirements of the Paris Climate Agreement are met, the structure of world energy in the coming decades must undergo radical changes as a result of the efficient use of all types of fuel.
It is necessary to avoid the emission of CO2; therefore, there are calls to abandon coal completely. Some experts, in particular – RAS Corresponding Member Vladimir Klimenko, argue: under any scenarios of energy development, even if we stop burning fossil fuel altogether, global warming won’t stop. Therefore, there is no need to make any large-scale abrupt changes in the energy structure. This would just be a disaster for the entire economy. The near future of the energy sector is fossil fuel and associated steam and gas technologies, deep processing of coal, and nuclear power. The more distant future is renewable energy sources. But it is possible to significantly increase the efficiency of all types of fuel with the help of hydrogen technologies in Russia – a country with vast territories, huge reserves of natural gas (from which additional “blue” energetic hydrogen can be produced), and great potential for renewable energy sources. Price factors also support the development of hydrogen technologies in Russia. We have a low cost of gas, which accounts for 45 to 70% of the cost of hydrogen production. In addition, we have cheap electricity. So, in Russia, hydrogen technologies will be more cost-effective than, for example, in Europe.
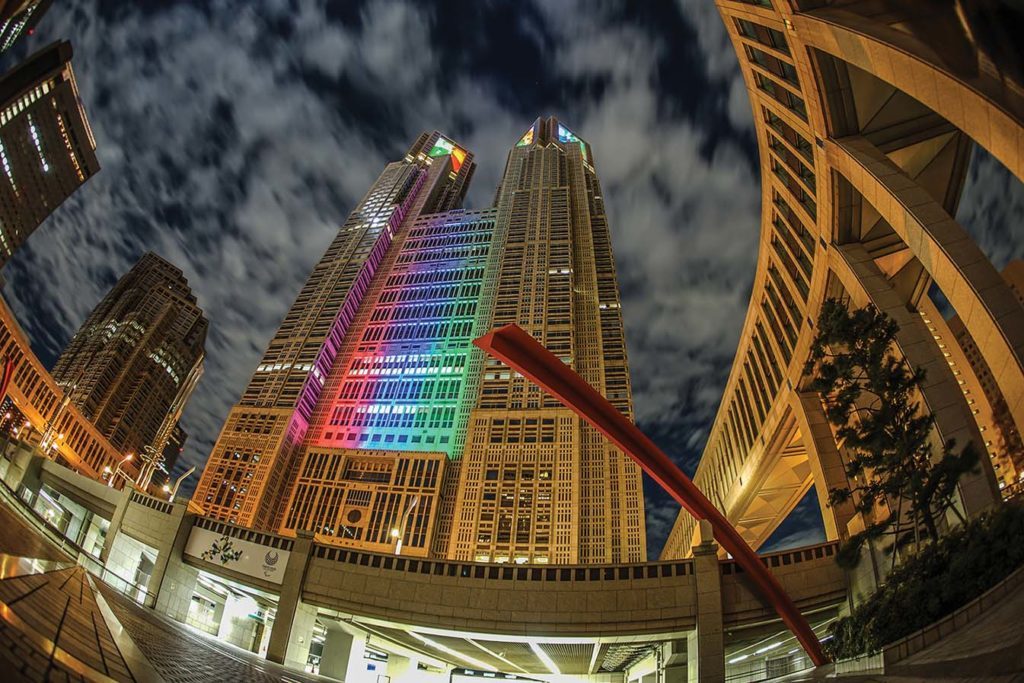
Source: kanzilyou / depositphotos.com
Hydrogen is a promising niche. Many countries have already adopted hydrogen strategies and roadmaps. In Europe, they are making quite serious financial investments in this direction.
According to estimates from the Hydrogen Council and the Hydrogen Analysis Resource Center, the global hydrogen market could be about $2.5 trillion by 2050. In physical terms, in the global energy balance, the share of hydrogen could reach about 18% of final energy demand, which would reduce CO2 emissions by 6 Gt per year. At the same time, in the transport sector, by 2050, the share of hydrogen cars will be from 15 to 20% (the number of hydrogen-fueled cars will be about 400 million, hydrogen-fueled trucks – 15-20 million and about 5 million hydrogen-fueled buses). To achieve these indicators, 20-25 billion dollars of investments will be required annually until 2030. For comparison: investments in the oil and gas industry – even during the crisis – amounted to about $60 billion.
Features of the physical and chemical properties of hydrogen will require the development of standards for equipment, production, transportation, storage and use. Metrology and the technological basis of physical and chemical safety will become very important elements of the future measurement infrastructure and quality and safety control systems. To ensure the above, it will be necessary to develop scientifically-grounded and legally-enshrined measurement methods, as well as internationally-recognized regulations, rules and standards. All of these measures are necessary to ensure safety, since negative examples and accidents will be a serious obstacle to the development of hydrogen technologies, causing political damage to the nascent hydrogen industry.
Establishing international business relations with foreign companies in the field of the hydrogen economy will contribute to the accelerated development of the national hydrogen market and strengthen the positions of Russian companies in this field. The creation of components for the production, application and transportation of hydrogen will be a serious driver for the development of a complete production chain.
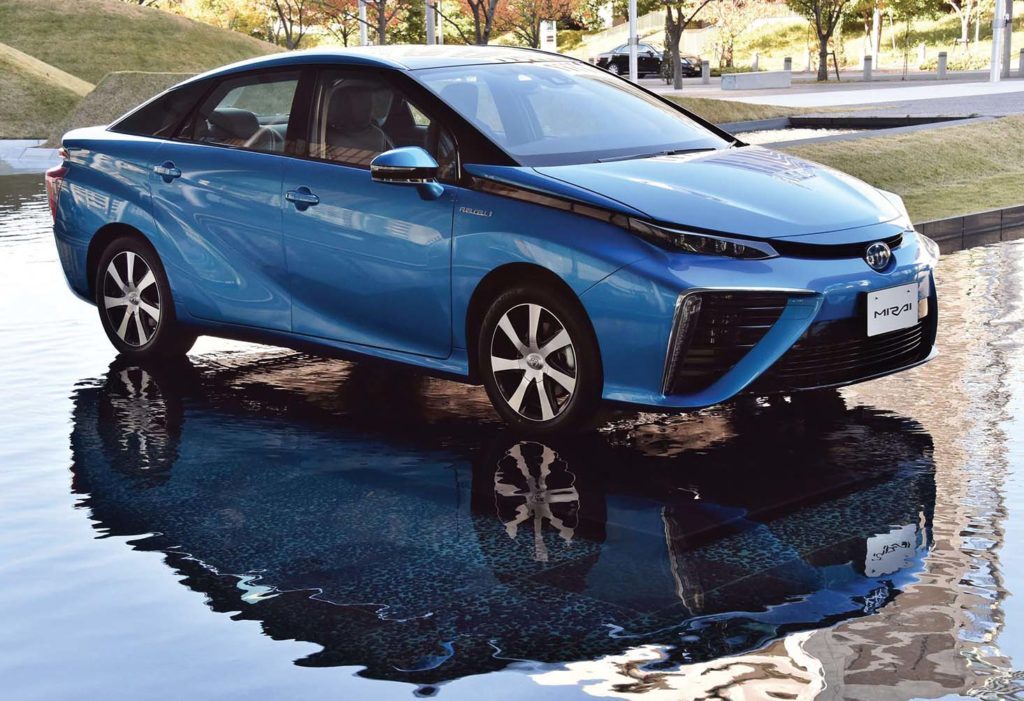
Source: allinallnews.com
Analysis of government programs for the development of hydrogen technologies
There are already 23 international hydrogen consortia in the world. Hydrogen valleys have sprung up in Germany, the Netherlands and Japan.
According to the adopted Energy Strategy until 2035, Russian plans to export 200 thousand tons of hydrogen by 2024, and ten times more by 2035. On the whole, the consumption and production of oil and gas will not fundamentally change for many years, so current resources (including the production of hydrogen) are quite sufficient.
The Ministry of Energy has developed a roadmap for the development of hydrogen energy in Russia until 2024. It stipulates that Gazprom and Rosatom will become the first producers of hydrogen in the country – in 2024, they are to launch pilot hydrogen plants, including those at nuclear power plants.
About 20 countries, including Japan, South Korea, Germany, China and the United States (primarily the state of California), are now actively developing the energy hydrogen market, building partnerships between the public and private sectors. A number of such partnerships are already in operation: H2Mobility Germany, H2Korea, the California Fuel Cell Partnership, and the Scandinavia Hydrogen Highway Partnership.
China plans to install up to 1,000 hydrogen fueling stations (HFS) by 2030, serving more than 1 million hydrogen vehicles (FCEV). By 2025, they also expect to transform the City of Wuhan into the country’s leading hydrogen hub. By 2025, the city will be home to the largest enterprises in the field of fuel cell production and more than 100 enterprises related to hydrogen energy. The number of HFS will be 30-100 stations. The volume of investments is estimated at about $1.7 billion.
In South Korea, according to the Hydrogen Analysis Resource Center, only 12 HFS were operating in 2018. However, thanks to the small territory of the country and the correct location of these fueling stations, drivers can cross the entire country by hydrogen car. South Korea’s Ministry of Industry, Trade and Energy has announced $2.3-billion plans to provide 16,000 hydrogen-fueled vehicles and build 310 fueling stations across the country by 2022. According to the five-year plan, businesses are expected to receive government support to develop fuel cell stacks and fuel cell storage containers, as well as tax incentives for hydrogen car drivers.
Japan is one of the leaders in investments in terms of government support, regulation and infrastructure to support the transition to hydrogen energy. The main goal is to improve air quality, significantly reduce emissions from the transport and industrial sectors and reduce dependence on imported fossil fuels. It was this country that produced the first commercially-manufactured car – the Toyota Mirai. In mid-2018, according to the Hydrogen Analysis Resource Center, Japan had 94 HFS, while Germany, which has the second-highest number of HFS, had 44 stations.
As outlined in the Strategic Roadmap for Hydrogen and Fuel Cells, Japan’s Ministry of Energy, Trade and Industry (METI) [1, 2] is coordinating a long-term strategy to accelerate the adoption of hydrogen vehicles, buses driven by hydrogen fuel cells, and hydrogen production plants. METI expects Japan’s annual hydrogen consumption to grow from 4000 tons in 2020 to 300 tons by 2030 and 5-10 million tons by 2050. According to the Strategic Roadmap for Hydrogen and Fuel Cells, the number of hydrogen cars should reach 40,000 by 2020, 180,000 by 2025, and about 800,000 by 2030. At the same time, it is planned to develop the network of fueling stations up to 160 stations by 2020 and about 320 stations by 2025 from the current 90 stations. It is expected that at least 1,200 fuel cell buses will be on the road by 2030.
Japan is actively introducing hydrogen technologies – back in 2014, it adopted a roadmap to build a “hydrogen-based society.” Japan is considering switching to hydrogen through imports from Brunei, Africa and Australia. For this purpose, Japanese companies such as Chiyoda Corporation, Kawasaki Heavy Industries, Iwatani, J-POWER and Marubeni are investing in hydrogen projects in Australia and Brunei.
Australia is a potential major hydrogen producer. In Australia, Japanese companies are cooperating with AGL Energy and Shell to create a $375-million liquefied hydrogen supply chain to Japan (the HESC project). According to the project, hydrogen will be produced from syngas, which will be obtained through the gasification of brown coal, while carbon capture and storage (CCS) technologies will be applied to ensure compliance with environmental standards. Extensive empty land plots to construct renewable energy facilities, favorable geographic locations to generate large amounts of solar and wind energy, and high investments in peak renewable energy production give Australia significant potential for hydrogen production. Investors’ interest in Australia as a potential supplier of hydrogen should be noted separately. At the moment, if the investment pays off, Australia could become the largest exporter not only of LNG but also of hydrogen.
In South Australia, Neoen, a French company recently operating in the field of renewable energy, announced plans to establish a hydrogen supercenter at Crystal Brook to export renewable hydrogen to Asia. A 50 MW hydrogen electrolyzer will be powered by a wind-and-solar plant with a total capacity of 300 MW, and a 400 MW/h storage unit will also be built. It is planned that the production of hydrogen could reach 20-25 tons per day. Neoen is already cooperating with Siemens and Hyundai to build a 1.25 MW low-capacity electrolyzer.
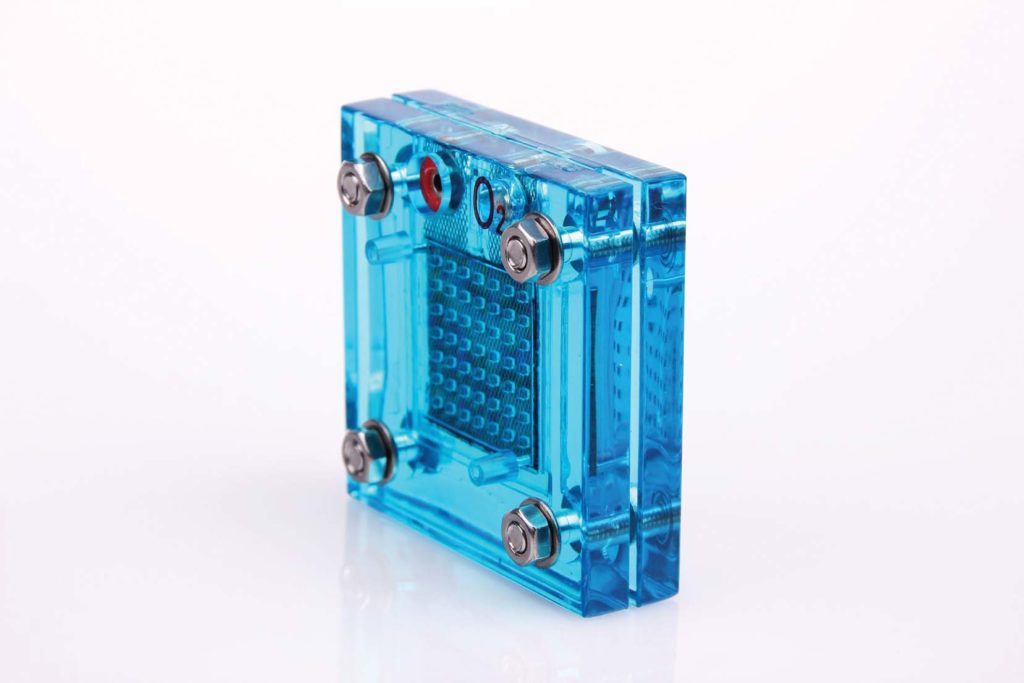
Source: flipboard.com
Hydrogen in Europe and the USA. The largest global market for hydrogen is going to become the European Union, which by 2050 intends to invest from 180 to 470 billion euros in the renewable hydrogen segment. There are 91 hydrogen buses in operation in European cities – up from 30 vehicles 5 years ago. It should be noted that the operating experience is very successful. Thus, according to the FCH JU (Fuel Cells and Hydrogen Joint Undertaking) partnership, which provides support for 67 hydrogen buses in Europe, there is interest from transport companies and in the coming years, their fleet should grow to 500 vehicles.
The Fuel Cells and Hydrogen Joint Undertaking finances pilot projects (for example, Don Quichote and HyBalance) for the production of hydrogen by water electrolysis based on wind and solar power plants, and tests new technologies for the thermolysis of water within the Hydrosol project, and new technologies for photoelectrolysis (ARTIPHYCTION and PECDEMO projects). The volume of investments on the part of FCH JU in the aforementioned projects alone has already reached about 30 million euros.
Overall, according to Hydrogen Europe, more than 200 projects in the field of hydrogen energy are currently being implemented in Europe.
In the United States, the State of California is the driving force behind the advancement of hydrogen vehicles. Thanks to state initiatives, by 2020, the number of HFS will have reached 62 stations. To date, the number of FCEVs has almost reached 5,000 – the largest hydrogen vehicle fleet in the world, and the number of operating fueling stations is 36. At the same time, the state government has set itself an extremely ambitious goal: by 2030, the number of vehicles should reach 1 million with 1000 HFS.
Today, the United States is the leader in terms of the number of hydrogen vehicles, and Japan is the leader in terms of fueling infrastructure. Major investments are being made in Europe – mainly in Germany and Scandinavia, and in China.
The German federal government is creating conditions for the production, transportation, application and further use of hydrogen. The National Hydrogen Strategy promotes innovative development and investment activity in the relevant areas. It defines the measures needed to achieve national climate change targets, to create new value chains in the German economy, and to further expand cooperation in the field of energy policy.
The plans are to stimulate the expansion of the market for hydrogen technologies (especially in the development and use of electrolyzers) in accordance with the energy turnaround policy. The German government expects hydrogen demand to rise to 90-110 TWh by 2030.
In order to partially meet the demand for hydrogen, it is necessary to create production facilities in Germany with a capacity of up to 5 GWh. This will provide for the possibility of hydrogen production in an amount of up to 14 TWh, which will require 20 TWh of “green” electricity. The government plans to analyze in detail the dynamics and structure of demand for “green” hydrogen to monitor the national hydrogen strategy.
Hydrogen is of great importance to the chemical and steel industries in Germany. In the near future, the industrial sector plans to use 55 TWh of hydrogen, whereas currently, the bulk of it is produced from fossil fuels. Recent expert estimates indicate that by 2050, the steel industry will need 80 TWh of hydrogen to achieve carbon neutrality. The production process for ammonia and petrochemicals will require an additional 22 TWh of hydrogen.
The expansion of the sales market for hydrogen and its by-products assumes the development of transport infrastructure.
Germany has a well-developed energy gas transportation system: a network of gas pipelines and gas storage facilities.
Hydrogen energy storage systems
Main types of storage systems. There are many different classifications of electric energy storage systems. The most convenient from the practical standpoint is the classification into electromagnetic and electrochemical, physical (mechanical), hydrogen and aluminum-hydrogen energy storage systems.
The former store electricity in superconducting coils or convert it into the chemical energy of substances, the latter convert it into mechanical energy.
Electrochemical energy storage devices include storage batteries, energy storage devices based on molecular capacitors, etc. All types of electrochemical storage devices are connected to the network through converters (inverters).
The physical storage of electricity mainly includes two types of complexes: kinetic energy storage devices (flywheels), gravitational energy storage devices (PSP). In [5, 6, 7, 8], a review of energy storage systems and an analysis of their characteristics are given.
Hydrogen energy storage systems consist of a hydrogen production system, fuel cells, and a communication and control system with an electric power system.
Fuel cells. A fuel cell (FC) is an electrochemical device that’s similar to a galvanic cell but different in that substances for the electrochemical reaction are supplied to it from the outside – in contrast to the limited amount of energy stored in a galvanic cell or battery. Fuel cells do not have the efficiency limitation that thermal machines do. In this regard, they can have a very high conversion factor of chemical energy into electrical energy.
The design of any fuel cell consists of two electrodes (cathode and anode) and an electrolyte layer between them – a medium that ensures the movement of ions from one electrode to the other and blocks the movement of electrons. In order to achieve higher rates of reaction, catalysts are often used in the electrodes. The functioning of fuel cells is supported through the supply of two components used to maintain the reaction – a fuel and an oxidizing agent. Depending on the type of fuel cell, gaseous hydrogen, natural gas (methane) and liquid hydrocarbon fuels (such as methyl alcohol) can be used as fuel. The oxygen in the air usually acts as an oxidizing agent, and some types of fuel cells can only work with pure oxygen.
Technological advances in the production of fuel cells have led to the fact that hydrogen can be used in fuel cell batteries for large and small power generation, heating and, of course, transport. Over the past 15 years, the cost of a hydrogen fuel cell has dropped from $275 to $55/kW – more than 5 times. The U.S. Department of Energy plans to reduce the cost of a fuel cell to $30/kW by 2022. Fuel cells do not convert the chemical energy of the fuel into thermal and mechanical energy, as in the traditional energy sector (Fig. 3). In this regard, the efficiency of fuel cells is significantly higher than that of traditional power plants and can be as high as 90%. There’s the possibility of the near-instant renewal of their energy resource – for that, it’s enough to install a new container (cartridge) with the fuel being used. The use of electrodes that are not consumed in the reaction process makes it possible to create fuel cells with very long service life. The high ecological purity of chemical fuel cells is noted. Only traditional fuels serve as a consumable for fuel cells, and the main reaction product is ordinary water. Replacing the currently used batteries and accumulators with fuel cells will significantly reduce the volume of waste to be processed, containing toxic and harmful substances for the environment.
Aluminum-hydrogen energy storage devices
Aluminum is very close to hydrogen (which is currently viewed as a prospective fuel) in terms of energy potential [9]. At the same time, aluminium is free of the drawbacks inherent in hydrogen (extremely low density of the gas and explosiveness). The storage and transportation of hydrogen give rise to a lot of safety-related issues. Moreover, there is thus far no simple and inexpensive method to produce hydrogen in massive amounts from renewable resources.
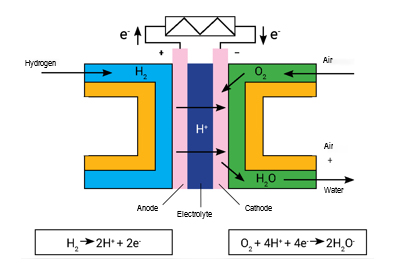
Aluminum is the most widespread metal in nature and the third most-common chemical element after oxygen and silicon. In normal conditions, aluminium is chemically inert. That said, the products of its oxidation can be reused to recover metal, so there is no need for a significant expansion of aluminum-containing mineral mining.
The Joint Institute for High Temperatures at the Russian Academy of Sciences (JIHT RAS, Moscow) has developed a series of air-aluminium fuel cells. Thus, aluminium can participate in the distribution of environmentally-friendly (as compared to fossil fuels) energy from renewable sources and NPPs and in regulating their generating capacity. In this case, the produced oxides are returned to the aluminium plant for recovery.
Hydrogen is a promising fuel of the future – including for use in fuel cells. Hydrogen, just like aluminium, can be delivered to the place of consumption and converted into useful electric and thermal energy.
Hydrogen can be obtained through the direct electrolysis of water by an electric current.
This is how the problem of storing electricity can be solved. This energy storage arrangement can be used to regulate the performance of power plants of the traditional type and those based on RES due to the higher flexibility of water electrolyzers as compared to aluminium electrolyzers, which require buffer storage because of their high sensitivity to changes in operating modes. However, there are serious restrictions on the transportation of hydrogen in cylinders because of fire and explosion hazards.
There’s the option of cryogenic hydrogen storage, but that’s not completely safe either and entails the costs associated with gas liquefaction and further losses during transportation due to evaporation. Quite common is the method of storing hydrogen in hydrides of intermetallic and metal-hydride compounds; however, its significant drawback is the low hydrogen capacity of these compounds (1-3%), high cost and the low number of hydrogenation/dehydrogenation cycles.
The traditional option of using diesel fuel, aside from its environmental impact, has a “power industry” disadvantage, i.e. the density of stored energy is less than in the case of aluminium. In addition, aluminium, as opposed to hydrogen and diesel fuel, is more convenient for transportation (not flammable, not fluidal, doesn’t volatilize).
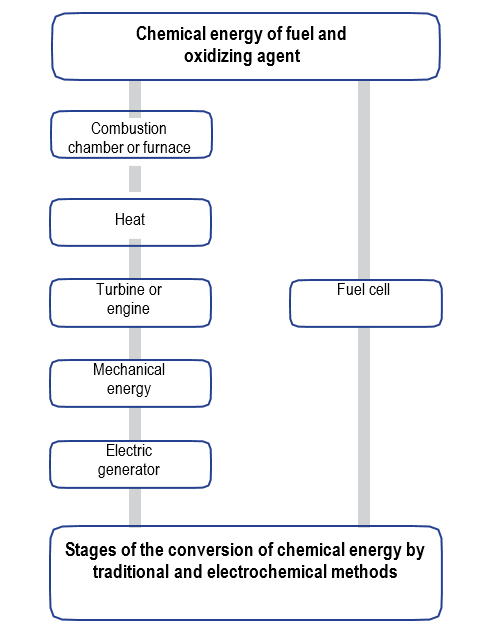
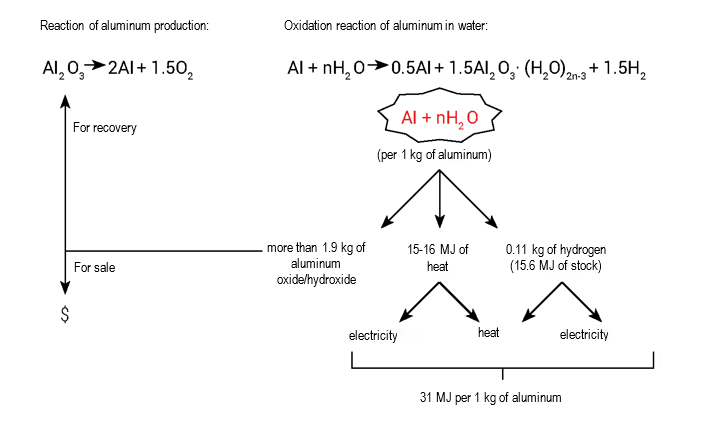
The developed technologies of aluminum-hydrogen energy can be applied both in the “hydrogen economy” of the future as an efficient and safe means of transporting hydrogen and stored energy, and as a supplement to existing energy systems in regions where there is no centralized gas network or local types of fuel. The use of aluminium to generate hydrogen and energy allows for reducing the environmental burden. The effectiveness of such plants is largely determined by the cost of raw materials and by-products of the reaction, as well as by the presence or absence of competitive solutions for centralized power supply to consumers.
Current state, problems and trends in the development of energy storage systems
Prospects for electricity storage technology. The new technological model of the electric power industry is characterized by the increased complexity of power systems associated with the emergence of a sizeable share of distributed generation and a new class of players – active consumers who combine the functions of energy consumption and production, along with the increased requirements of energy consumers in terms of the accessibility, quality and reliability of power supply.
It’s important to understand that systemic electric energy storage devices (SEESD) are one of the key elements of the electric power industry with an intelligent control system. Potential areas of energy storage application in the power system include: smoothing the irregularity of power generation and consumption (including smoothing the irregularity of RES-generation), voltage and frequency control, provision of standby capacity, emergency power supply to prevent the development of emergency situations in the system (with power system is landing and recover the power system after such emergencies, to ensure the emergency power supply of consumers. The particular importance of energy storage devices lies in the fact that they can perform the aforementioned functions simultaneously.
SEESDs can consume and output active power, and being connected with components of power electronics, they can process reactive power as well. Depending on the needs of the power system, SEESDs can provide frequency and voltage regulation, time shift between consumption and generation, power regulation at the output of RES + SEESDs systems and expand the capabilities of dispatch control. They can be designed for the needs of distributing and/or transmitting systems, for single-purpose or multipurpose use, or for the purposes of control at the consumer side.
Each energy storage technology is characterized by the capital expenses required for its implementation, as well as operating expenses. In general, some energy storage technologies are not currently cost-effective, and moving forward, we first and foremost need to reduce capital expenses. The cost of various SEESDs and benefits from their application largely depend on their structure in terms of discharge power.
The required power of energy storage systems is about 30 GW. The energy consumption of storage systems is about 15 GW/h. A preliminary analysis shows [11, 12] that the payback period for a system based on lithium-ion batteries is 6-7 years.
The payback period of the system based on hydrogen and aluminum-hydrogen energy storage is 4-6 years, due to the localization of disturbances in the active and reactive power of loads, the stable quality of electric power, fuel savings at generating power plants, and an increase in the time-to-failure of generating equipment and the equipment of energy consumers.
Conclusion
- Hydrogen and aluminum-hydrogen energy storage systems are priority tasks in the development of hydrogen technologies and will provide a significant increase in the controllability, operational reliability and efficiency of electric power systems.
- The main goal of energy storage systems is the formation of new power-sector infrastructure free of the continuity restrictions of one type of electric processes, and in fact – a considerable extension of the type and form of power pools allowing for the integration of stand-alone, distributed and centralized systems, including new centers of generation and consumption, into a common power “system of systems.”
- According to experts, over the next 10 years, the market for energy storage systems will grow at average annual rates exceeding 30% with a trend towards the lower unit cost of the stored energy, where a special role is assigned to hydrogen technologies.